Balbharti Maharashtra State Board 12th Physics Important Questions Chapter 15 Structure of Atoms and Nuclei Important Questions and Answers.
Maharashtra State Board 12th Physics Important Questions Chapter 15 Structure of Atoms and Nuclei
Question 1.
What was Dalton’s atomic theory of chemistry?
Answer:
John Dalton (1766-1844), British meteorologist, in his atomic theory of chemistry (1808-1810) proposed the following postulates :
(1) Matter consists of very small indivisible particles called atoms.
(2) Each element consists of a characteristic kind of identical atoms. There are consequently as many different kinds of atoms as there are elements.
(3) When different elements combine to form a compound, the smallest unit of the compound consists of a definite number of atoms of each element. These ‘compound atoms’ are now called molecules.
(4) In chemical reactions, atoms are neither created nor destroyed, but only rearranged.
Question 2.
Explain Thomson’s model of the atom. What are its drawbacks?
Answer:
The first model of the atom with a sub-structure was put forward in 1898 by Sir J.J. Thomson (1856-1940), a British physicist. According to this model, an atom consists of a sphere with a uniform distribution of positive charge and electrons embedded in it such that the atom is electrically neutral and stable.
Drawbacks : This model, known as the plumpudding model, failed to account for the observed scattering of α-particles and spectra of various elements.
[Note : It can be shown that the Thomson atom cannot be stable.]

Question 3.
Who suggested the famous a-particle scattering experiment? Why?
Answer:
Sir Ernest Rutherford (1871-1937), New Zealand- British physicist, following his pioneering work on radioactivity and properties of a-particles, had noted that a narrow stream of α-particles gets somewhat broadened or scattered on passing through a thin metal foil or mica sheet. Since most of the α-particles remained undeviated, this suggested that atoms could not be solid spheres as proposed in Thomson’s model and kinetic theory of gases. To probe into the effects of the distribution of an atom’s mass and charge on the a-particles, he suggested his collaborator Geiger and the latter’s student Marsden to see if any α-particles are scattered through a large angle.
Question 4.
With the help of a neat labelled diagram, describe the Geiger-Marsden experiment.
Answer:
The Geiger-Marsden a-scattering (or gold foil) experiment (1908) : Geiger and Marsden made a stream of a-particles strike a very thin gold foil about 40 jum thick. Their apparatus is shown schematically in figure.
Apparatus: A radium compound, an intense source of a-particles, was placed in the lead enclosure B, provided with a small hole. The stream of α-particles was collimated by lead bricks. The number of particles scattered through each angle θ were counted by a rotatable detector. The detector consisted of a small zinc sulphide screen S at the focus of a low power microscope M. Each incidence produced a scintillation-a momentary pinpoint of fluorescence. These scintillations were observed and counted using the microscope.
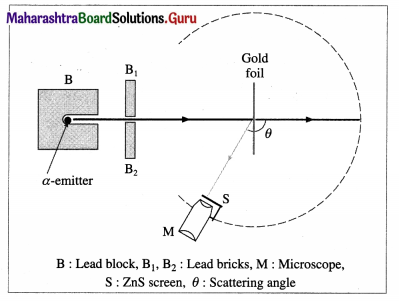
Geiger-Marsden experiment of scattering of a-particles by a gold foil
Observations: Most of the α-particles passed through the foil almost undeviated, with less than 0.2% deflected by more than 1°. Still smaller fractions were found to be deflected by 90° or more, sometimes almost straight back towards the source.
Rutherford quantitatively accounted for the distribution of small and large angle scattering by considering each scattering to be a single collision of an a-particle with a positive ‘central charge’ Ne concentrated at a point. Since the probability of an a-particle coming very close to such a point charge was small, this explained the very small number of a-particles deflecting through large angles.
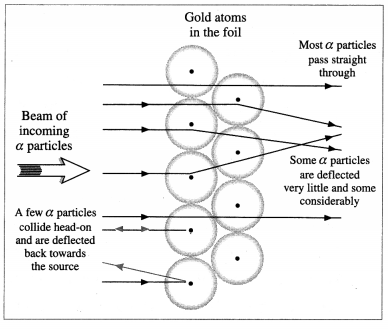
Scattering of a-particles by a gold foil
[Notes : (1) Hans Wilhelm Geiger (1882-1945), German physicist and Sir Ernest Marsden (1889-1970), English-New Zealand physicist. (2) In 1908 and 1909, they conducted a series of a-scattering experiments with gold and silver foils of different thicknesses and a thick platinum plate. Rutherford reported (in 1911) that “about 1 in 20000 were turned through 90° on passing through a gold foil about 40 nm thick.” The number ‘1 in 8000’ was reported (in 1909) by Geiger and Marsden for reflection off a thick platinum plate ‘at large angle.]

Question 5.
Explain Rutherford’s model of the atom.
Answer:
Rutherford’s model of the nuclear atom (1911) :
(1) An atom has a very small nucleus which contains all the positive charge and almost all the mass of the atom.
(2) The nuclear size (radius about 10-14 m) is very small compared to the atomic size (radius about 10-10m), about 10000 times smaller.
(3) Electrons revolve in circular orbits around the nucleus. The electrostatic force (Coulomb force) of attraction between the positively charged nucleus and the negatively charged electron is the centripetal force required for the orbital motion of the electron.
(4) Since an atom as a whole is electrically neutral, the positive charge on the nucleus must be equal to the total negative charge of all the orbiting electrons.
As this model resembles the solar system, it is known as the planetary atom model.
Question 6.
Solve the following :
(1) An a-particle having a kinetic energy of 8 MeV is projected directly toward the nucleus of an atom of \(\begin{gathered}
208 \\
82
\end{gathered}\)Pb. Find the distance of closest approach.
(e = 1.6 × 10-19 C, ε0 = 8.85 × 10-12 C2/N∙m2)
Solution :
Data : e = 1.6 × 10-19 C, Z(Pb) = 82, ε0 = 8.85 × 10-12 C2/N∙m2, (KE)α = 8 MeV
If d is the distance of closest approach, we must have, by the principle of conservation of energy, initial kinetic energy of the a-particle = potential energy of the a-particle when it is at the distance d from the centre of the nucleus of
\(\begin{gathered}
208 \\
82
\end{gathered}\) Pb.
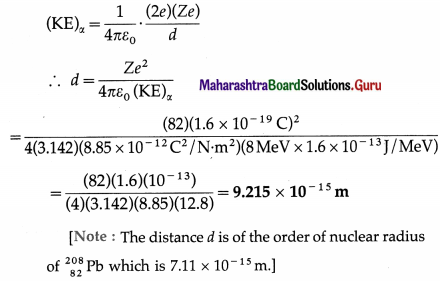
(2) An α-particle projected directly toward the nucleus of an atom of \(\begin{gathered}
208 \\
82
\end{gathered}\)Pb comes to rest momentarily at the surface of the nucleus. Find the initial kinetic energy and speed of the α-particle.
[m(α) = 6.68 × 10-27 kg, r1(α) = 1.8 × 10-15 m, r2(Pb) = 7.11 × -15 m, e = 1.6 × 10-19 C, \(\frac{1}{4 \pi \varepsilon_{0}}\) = 9 × 109 N-m2/C2]
Solution:
For reference, see the solved problem (1) above.
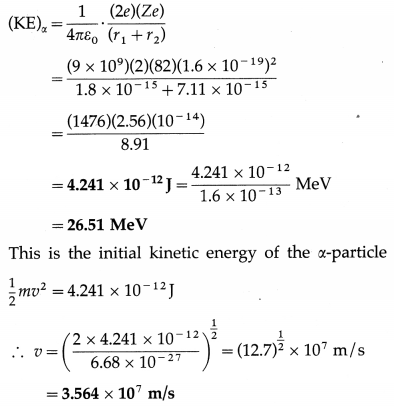
This is the initial speed of the α-particle.
[Note : 3.564 × 107 m/s is about 12% of the speed of light in vacuum.]

Question 7.
What is the emission spectrum of a substance? Explain in brief.
Answer:
The emission spectrum of a substance is the distribution of electromagnetic radiations emitted by the substance when it is heated, or bombarded by electrons, or ions or photons. The distribution is arranged in order of increasing (or decreasing) frequency (or wavelength) and is characteristic of the substance unless the temperature of the substance is very high when the distribution is continuous. The spectrum may be a line spectrum or band spectrum. The intensities corresponding to different frequencies are different.
[Note : The absorption spectrum is formed by absorption of electromagnetic radiation when the substance is exposed to radiation of all frequencies.]
Question 8.
State and explain the formula that gives wavelengths of lines in the hydrogen spectrum.
Answer:
Formula : \(\frac{1}{\lambda}=R\left[\frac{1}{n^{2}}-\frac{1}{m^{2}}\right]\), where λ is the wavelength of a line in the hydrogen spectrum, R is a constant, now called the Rydberg constant, and n and m are integers with n = 1,2,3,… and m = n + 1, n + 2, n + 3,
For a fixed value of n, λ decreases as m increases and has minimum value as m → ∞ λ = \(\frac{n^{2}}{R}\) as
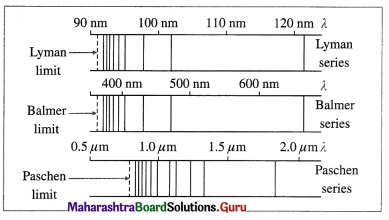
Lyman, Balmer and Paschen series in the hydrogen spectrum (For reference only)
[Note : A line spectrum is atomic in origin. It is a signature of the element, i.e., we can determine the elements present in a mixture of elements by studying the line spectrum of the mixture.]
Question 9.
State the equations corresponding to Bohr’s atomic model.
Answer:
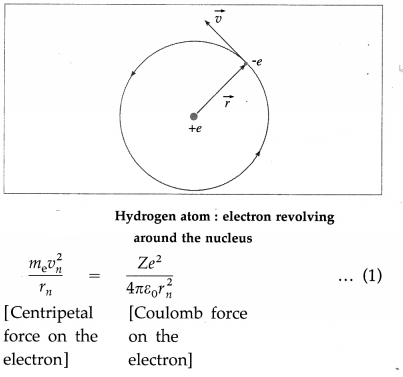
Here, me is the mass of the electron, e is the electron charge, Z is the atomic number of the atom, rn is the radius of the nth stable orbit, vn is the speed of the electron in the nth orbit and ε0 is the absolute premittivity of free space. In hydrogen, Z = 1.
me vn rn = n\(\frac{h}{2 \pi}\) …………… (2)
[Angular momentum of the electron]
where n ( = 1, 2, 3, …) is the positive integer, called the principal quantum number, and h is Planck’s constant, n denotes the number of the orbit.
Em – En = hv …………. (3)
Here, Em is the energy of the electron in the mth orbit, En is the energy of the electron in the nth orbit (m > n), hv is the energy of the photon emitted and v is the frequency of the electromagnetic radiation emitted.
[Note : Niels Bohr (1885-1962), Danish theoretical physicist, made significant contribution to atomic and
nuclear physics.]

Question 10.
What is the minimum angular momentum of the electron in an hydrogen atom?
Answer:
\(\frac{h}{2 \pi}\).
Question 11.
Which physical quantity of an atomic electron has the dimensions same as that of h?
Ans.
Angular momentum.
Question 12.
What is meant by a stationary orbit?
Answer:
In the Bohr model of the hydrogen atom, a stationary orbit refers to any of the discrete allowed orbits such that the electron does not radiate energy while it is in such orbits.
Question 13.
Derive an expression for the linear speed of an electron in a Bohr orbit. Hence, show that it is inversely proportional to the principal quantum number.
Answer:
Consider an electron revolving in the nth Bohr orbit around the nucleus of an atom with the atomic number Z. Let m and – e be the mass and charge of the electron, r the radius of the orbit and v the linear speed of the electron.
According to Bohr’s first postulate, centripetal force on the electron = electrostatic force of attraction exerted on the electron by the nucleus.
∴ \(\frac{m v^{2}}{r}=\frac{Z e^{2}}{4 \pi \varepsilon_{0} r^{2}}\) ………….. (1)
where ε0 is the permittivity of free space.
∴mv2 = \(\frac{Z e^{2}}{4 \pi \varepsilon_{0} r}\) ………….. (2)
According to Bohr’s second postulate, the orbital angular momentum of the electron is quantized :
mvr = \(\frac{n h}{2 \pi}\) ………… (3)
where h is Planck’s constant and n is the principal quantum number which takes integral values 1, 2, 3, …, etc.
∴ r = \(\frac{n h}{2 \pi m v}\)
Substituting this expression for r in Eqn (2), we get,
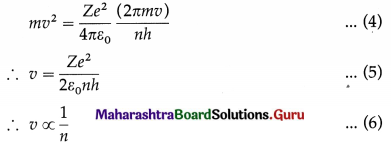
as Z, e, ε0 and h are constants.
[Note : In this topic, unless stated otherwise, m = me, r = rn, and v = vn.]

Question 14.
What is the angular momentum of the electron in the third Bohr orbit in the hydrogen atom ?
[\(\frac{h}{2 \pi}\) = 1.055 × 10-34 kg∙m2/s
Answer:
Angular momentum, L = mvr = \(\frac{nh}{2 \pi}\)
For n = 3, L = 3(\(\frac{h}{2 \pi}\)) = 3 (1.055 × 10-34)
= 3.165 × 10-34 kg∙m2/s
Question 15.
Derive an expression for the radius of the nth Bohr orbit in an atom. Hence, show that the radius of the orbit is directly proportional to the square of the principal quantum number.
Answer:
Consider an electron revolving in the nth orbit around the nucleus of an atom with the atomic number Z. Let m and – e be the mass and charge of the electron, r the radius of the orbit and v the linear speed of the electron.
According to Bohr’s first postulate, centripetal force on the electron = electrostatic force of attraction exerted on the electron by the nucleus.
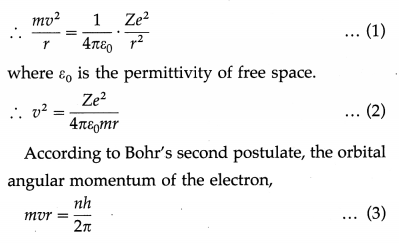
where h is Planck’s constant and n is the principal quantum number which takes integral values 1, 2, 3, …… etc.
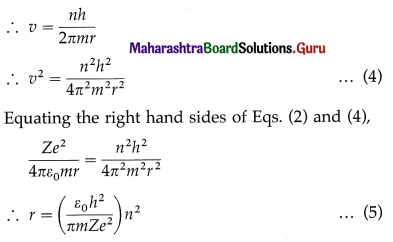
Since, ε0, h, Z, in and e are constants, it follows that r ∝ n2, i.e., the radius of a Bohr orbit of the electron in an atom is directly proportional to the square of the principal quantum number.
Question 16.
The radius of the first Bohr orbit in the hydro gen atom is 0.5315 Å. What is the radius of the second Bohr orbit in the hydrogen atom?
Ans.
In the usual notation,
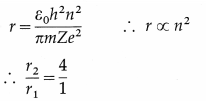
∴ r2 = 4r1 = 4 × 0.5315 = 2.125 Å is the required radius.
[Note : r1 is also denoted by a0.]
Question 17. Show that the angular speed of an electron in the nth Bohr model is ω = \(\frac{\pi m e^{4}}{2 \varepsilon_{0}^{2} h^{3} n^{3}}\) and the corresponding frequency of the revolution of the electron is f = \(\) .
Answer:
The radius of the nth Bohr orbit is
r = \(\frac{\varepsilon_{0} h^{2} n^{2}}{\pi m Z e^{2}}\) ………….. (1)
and the linear speed of an electron in this orbit is
v = \(\frac{Z e^{2}}{2 \varepsilon_{0} n h}\) ………….. (2)
where ε0 ≡ permittivity of free space, h ≡ Planck’s constant, n ≡ principal quantum number, m ≡ electron mass, e ≡ electronic charge and Z ≡ atomic number of the atom.
Since angular speed ω = \(\frac{v}{r}\), then from Eqs. (1) and (2) we get,

which gives the required expression for the angular speed of an electron in the nth Bohr orbit.
From Equestion (3), the frequency of revolution of the electron,
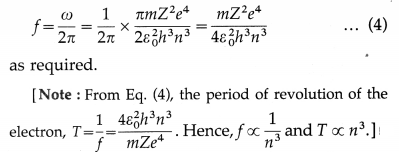

Question 18.
The angular speed of an electron in the first orbit in H-atom is 4.105 × 1016 rad/s. Find the angular speed of the electron in the second orbit.
Answer:

= 5.131 × 1015 rad/s This is the required quantity.
Question 19.
The frequency of revolution of the electron in the second Bohr orbit in the hydrogen atom is 8.158 × 1014 Hz. What is the frequency of revolution of the electron in the fourth Bohr orbit in the hydrogen atom ?
Ans.
In the usual notation,
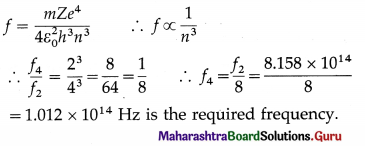
Question 20.
Show that the energy of the electron in the nth stationary orbit in the hydrogen atom is
En = -Rch/n2.
Answer:
The energy of the electron in the nth stationary orbit in the hydrogen atom is
En = \(-\frac{m e^{4}}{8 \varepsilon_{0}^{2} h^{2} n^{2}}\)
and the Rydberg constant is
R = \(\frac{m e^{4}}{8 \varepsilon_{0}^{2} h^{3} c}\)
where m = mass of electron, e = electronic charge, h = Planck’s constant, c = speed of light in free space and i, = permittivity of free space.
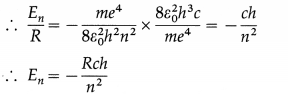
Question 21.
State the limitations of Bohr’s atomic model.
Answer:
Limitations of Bohr’s atomic model:
- The model cannot explain the relative intensities of spectral lines even in the hydrogen spectrum.
- The model cannot explain the atomic spectra of many-electron atoms of higher elements.
- The model cannot account for the Zeeman effect and Stark effect (fine structure of spectral lines as revealed in the presence of strong magnetic field and electric field, respectively).
[Note : In 1896, Pieter Zeeman (1865-1943), Dutch physicist, discovered the splitting of spectral lines by magnetic field. In 1913, Johannes Stark (1874-1957), German physicist, discovered the splitting of spectral lines by electric field.]
Question 22.
Draw a neat, labelled energy level diagram for the hydrogen atom. Hence explain the different series of spectral lines for hydrogen.
Answer:
According to Bohr’s model of the hydrogen atom, an atom exists most of the time in one of a number of stable and discrete energy states. The various states arranged in order of increasing energy constitute the energy level diagram of the atom, as shown in below figure for the hydrogen atom. Here, the higher (less negative) energies are at the top while the lower (more negative) energies are toward the bottom.
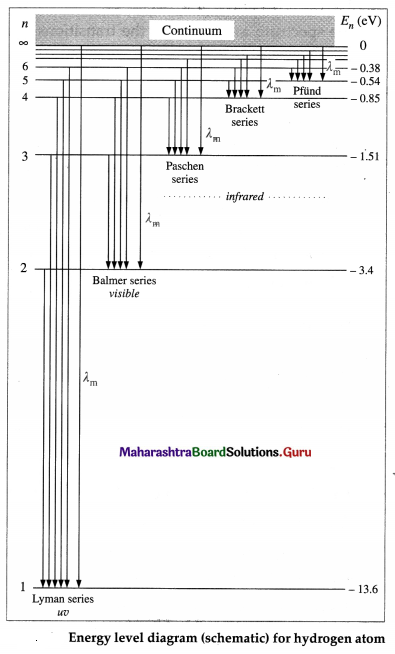
According to Bohr’s theory, electromagnetic radiation of a particular wavelength is emitted when there is a transition of the electron from a higher energy state to a lower energy state. Let the quantum number n = m represent a higher energy state and n = n represent a lower energy state (m > n). The formation of the different series of spectral lines is explained from the energy level diagram.
(1) Lyman series : This series in the far UV region of the spectrum arises due to the transitions of the electron to n = 1 from m = 2, 3, 4, …, etc. The wavelengths (λ) of the spectral lines in this series are given by
\(\frac{1}{\lambda}=R\left(\frac{1}{1^{2}}-\frac{1}{m^{2}}\right)\) (m = 2, 3, 4, ……….,∞)
where R is the Rydberg constant.
(2) Balmer series : This series in the visible region of the spectrum arises due to the transitions to n = 2 from m = 3, 4, 5, …, etc. The wavelengths (λ) of the spectral lines in this series are given by
\(\frac{1}{\lambda}=R\left(\frac{1}{2^{2}}-\frac{1}{m^{2}}\right)\) (m = 3, 4, 5, …. ∞)
(3) Paschen, Brackett and Pfiind series : These three series in the infrared region of the spectrum arise due to the transitions to n = 3, 4 and 5, respectively from m=n + 1, n + 2, etc. The wavelengths (λ) of the lines are given by
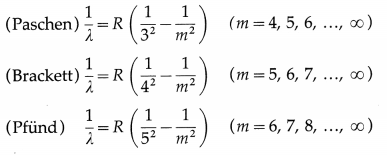
In each series, the smallest quantum of radiation (smallest frequency, longest wavelength) arises from the transition from m = n +1 to n, and the largest quantum (highest frequency, shortest wavelength — short wavelength limit or series limit) is for the transition from m = ∞ to n.
[Notes :
(1) The lines in the Lyman and Balmer series, beginning with the longest wavelength, are labelled with the Greek letters α, β, γ, δ, ε, …. Thus, the lines in the Lyman series are called Lα, Lβ, Lγ, Lδ … lines while those in the Balmer series are called Hα, Hβ, Hγ, Hδ, … lines. Thus, the Lα, line has a wavelength λ, where
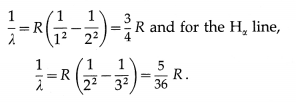
(2) The first four prominent hydrogen lines (Hα, Hβ, Hγ, Hδ) lie in the visible region and were discovered in the solar spectrum by Anders Angstrom (the first three in 1862 and the fourth in 1871); he also measured their wavelengths to high accuracy.
Johann Jakob Balmer (1825 – 98), Swiss mathema-tician, discovered in 1884 that their wavelengths fitted
the relation λ = \(\frac{B m^{2}}{m^{2}-4}\), where m has integral values 3, 4, 5 and 6 for successive lines and B here is a constant equal to 3645.6 Å. This is Balmer’s formula; originally empirical, it pointed to the need to find an explanation. This led through Rydberg’s work to Bohr’s theory.
(3) Lyman series was discovered between 1906-14, Paschen series in 1908, Brackett series in 1922 and Pfiind series in 1924. A sixth, and the last of the named series in the hydrogen spectrum, is the Humphreys series which results from transitions to n = 6 from m = 7, 8, 9, … etc. The Paschen series lies in the near-infrared region while Pfiind and Humphreys series lie in the far-infrared region.
Further series are unnamed but follow the same pattern. Their lines are increasingly faint showing that they correspond to increasingly rare atomic events.]

Question 23.
Obtain the expressions for longest and shortest wavelengths of spectral lines in ultraviolet region for hydrogen atom.
Answer:
For hydrogen,
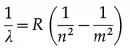
where R is the Rydberg constant, and m and n are the principal quantum numbers of the initial and final energy states. The Lyman series in the far UV region of the spectrum arises due to the transitions of the electron to n = 1 from,m = 2, 3,4, …, etc.
For the longest wavelength λLα in the Lyman series, m = 2.
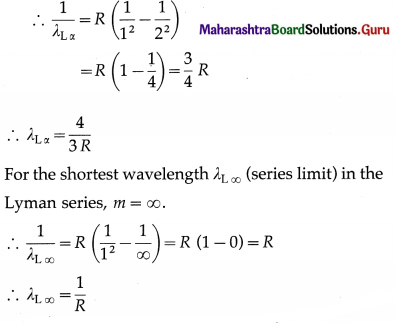
Question 24.
How many spectral series are possible in the hydrogen spectrum?
Answer:
Infinite. The last (sixth) of the named series in the hydrogen spectrum is the Humphreys series which results from transitions to nf = 6 from ni = 7, 8, 9, … etc. in the far-infrared region. Further series are unnamed but follow the same pattern. Their lines are increasingly faint showing that they correspond to increasingly rare atomic events.
[Note : There are infinite number of lines in each series. The spacing between adjacent lines decreases with decreasing wavelength, converging to the so-called series limit or short-wavelength limit.]
Question 25.
The energy of the electron in the first Bohr orbit in the hydrogen atom is -13.6 eV. What is its energy in the second and third Bohr orbit?
Answer:
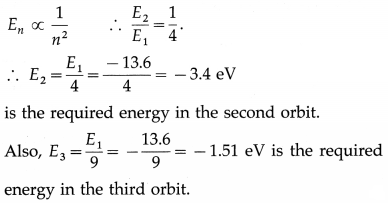
Question 26.
The potential energy of the electron in the first Bohr orbit in the hydrogen atom is -27.2 eV. What is its kinetic energy and binding energy in the same orbit?
Answer:
Kinetic energy = –\(\frac{\text { potential energy }}{2}=-\frac{27.2}{2}\)
= 13.6 eV and
binding energy = – total energy – (potential energy + kinetic energy)
= – (-27.2 + 13.6) = 13.6 eV

Question 27.
Obtain the ratio of the longest wavelength of spectral line in the Paschen series to the longest wavelength of spectral line in the Brackett series.
Answer:
The Bohr formula for the wave number of a spectral line in the hydrogen spectrum is
\(\bar{v}=\frac{1}{\lambda}=R\left(\frac{1}{n^{2}}-\frac{1}{m^{2}}\right)\)
where is the wavelength of the emitted radiation when the electron jumps from a higher energy state of principal quantum number m to a lower energy state of principal quantum number n. R is the Rydberg constant.
The Paschen series and Brackett series of spectral lines arise due to the transitions to n = 3 and m = 4, respectively. The longest wavelength lines (λPα and λBx) in these series arise due to the transitions from m =4 and m = 5, respectively.
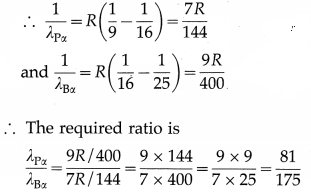
Question 28.
Obtain the ratio of the wavelength of the Hα line to the wavelength of the Hγ. line in the Balmer series.
Ans.
The Bohr formula for the wave number of a spectral line in the hydrogen spectrum is
\(\bar{v}=\frac{1}{\lambda}=R\left(\frac{1}{n^{2}}-\frac{1}{m^{2}}\right)\)
where λ is the wavelength of the emitted radiation when the electron jumps from a higher energy state of principal quantum number m to a lower energy state of principal quantum number n. R is the Rydberg constant.
The Balmer series of spectral lines arises due to the transitions to n = 2. The Hα and Hγ lines in this series arise due to the transitions from m = 3 and 5, respectively.
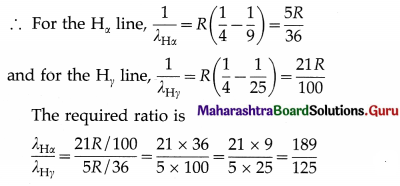
Question 29.
On the basis of the de Broglie hypothesis, obtain Bohr’s condition of quantization of angular momentum.
Answer:
By the de Broglie equation, the wavelength associated with an electron having momentum p = mv is
λ = \(\frac{h}{p}=\frac{h}{m v}\) ……….. (1)
In a hydrogen atom in its ground state, the de Broglie wavelength associated with the electron is the same as the circumference of the first Bohr orbit. Therefore, the electron orbit in a hydrogen atom in its ground state corresponds to one complete electron wave joined on itself.
Thus, a stationary orbit can be intepreted as one which can accommodate an integral number of de Broglie wavelength so that the associated matter wave will be in phase with itself and constructive interference will allow a standing wave along the orbit.
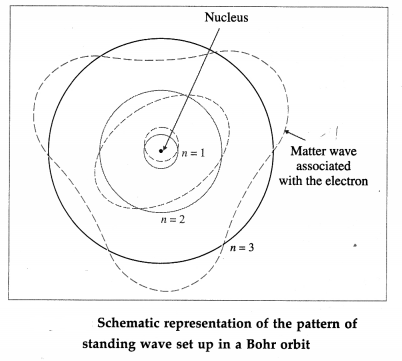
Therefore, for a stationary Bohr orbit of circumference 2πr,
2πr = nλ
where n is a positive integer.
∴ 2πr = \(\frac{n h}{m v}\) …………. [From Eqn (1)]
∴ Angular momentum, L = mvr = n(\(\frac{h}{2 \pi}\))
which is just the Bohr condition of angular momentum quantization for stable or allowed orbits.
30. Solve the following :
Data: e = 1.6 × 10-19 C, me = 9.1 × 10-31 kg, h = 6.63 × 10-34 J∙s, c = 3 × 108 m/s. I eV = 1.6 × 10-19 J, ε0 = 8.85 × 10-12 F/m, R = 1.097 × 107 m 1, 1/4πε0 = 9 × 109 N∙m2/C2
Question 1.
The radius of the first orbit of the electron in a hydrogen atom is 0.53 Å. Find the centripetal force acting on the electron.
Solution:
Data : r = 5.3 × 10-11 m
The centripetal force on the electron = the electrostatic force between the proton and electron
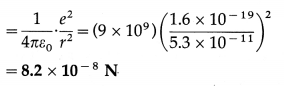
Question 2.
Calculate the angular momentum of the electron in the third Bohr orbit of hydrogen atom.
Solution:
Data: n = 3
The angular momentum, L = \(\frac{n h}{2 \pi}\)
= \(\frac{3\left(6.63 \times 10^{-34}\right)}{2(3.142)}\) = 3.165 × 10-34 kg.m2/s

Question 3.
Calculate the potential energy of the electron in the second Bohr orbit of hydrogen atom in electron volt. The radius of the Bohr orbit is 2.12 Å.
Answer:
Data : r = 2.12 × 10-10 m
The potential energy of the electron
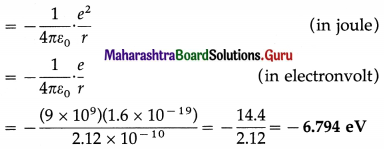
Question 4.
Calculate the radius of the first Bohr orbit in the hydrogen atom. [ε0 = 8.85 × 10-12 C2/N∙m2 e = 1.6 × 10-19 C, m(electron) = 9.1 × 10-31 kg, h = 6.63 × 10-34 J∙s]
Solution:
Data : ε0 = 8.85 × 10-12 C2/N∙m2 e = 1.6 × 10-19 C,
h = 6.63 × 10-34 J∙s, m = 9.1 × 10-31 kg
The radius of a Bohr orbit,
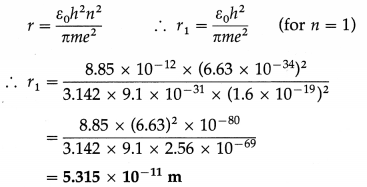
This is the radius of the third Bohr orbit in a hydrogen atom.
Question 5.
Find the ratio of the diameter of the first Bohr orbit to that of the fourth Bohr orbit in a hydrogen atom.
Solution:
The radius of a Bohr orbit,
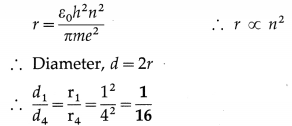
Question 6.
Calculate the frequency of revolution of the electron in the second Bohr orbit of the hydrogen atom. The radius of the orbit is 2.14 Å and the speed of the electron in the orbit is 1.09 × 106 m/s.
Solution:
Data: r = 2.14Å = 2.14 × 10-10 m, v = 1.09 × 106 m/s
v = ωr = (2πf)r
∴ The frequency of revolution of the electron in the second Bohr orbit,
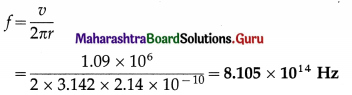
Question 7.
The speed of the electron in the first Bohr orbit (in H atom) of radius 0.5 Å is 2.3 × 106 m/s. Calculate the period of revolution of the electron in this orbit.
Solution:
Data: r = 0.5 Å = 5 × 1011 m, v = 2.3 x 106 m/s
Period of revolution of the electron,
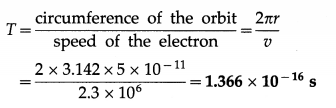

Question 8.
The energy of the electron in the ground state of the hydrogen atom is -13.6 eV. Find its KE and PE in the same state.
Solution:
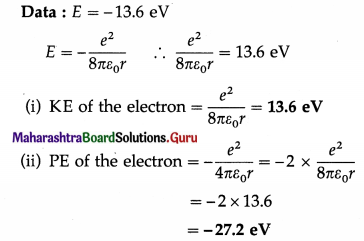
Question 9.
An electron is orbiting in the 3rd Bohr orbit in H atom. Calculate the corresponding ionization energy, if the ground state energy is – 13.6 eV.
Solution:
Data : E1 = -13.6 eV, n1 = 1, n3 = 3, E∞ = 0 eV
The energy of the electron in the nth Bohr orbit,
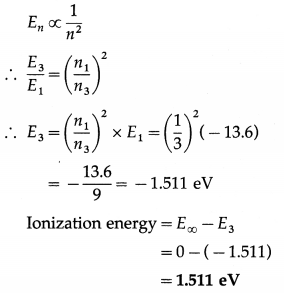
Question 10.
Find the energy of the electron in the fifth Bohr orbit of the hydrogen atom. [Energy of the electron in the first Bohr orbit -13.6 eVI
Solution:
Data : E1 = -13.6 eV
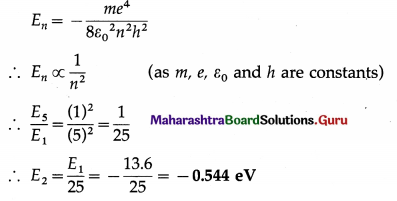
Question 11.
Calculate the energy of the electron in the ground state of the hydrogen atom. Express it in joule and in eV.
[melectron = 9.11 × 10-31 kg, e = 1.6 × 10-19 C, h = 6.63 × 10-34 J∙s, ε0 = 8.85 × 10-12 C2/N∙m2]
(3 marks)
Solution:
Data: m = 9.11 × 10-31 kg, e = 1.6 × 10-19 C, h = 6.63 × 10-34 J∙s, ε0 = 8.85 × 10-12 C2/N∙m2
The energy of electron in nth Bohr orbit is
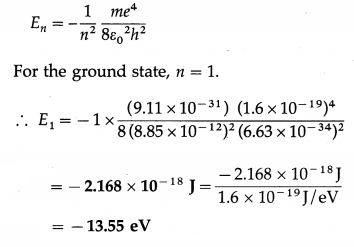

Question 12.
The energy of the electron in an excited hydrogen atom is – 0.85 eV. Find the corresponding angular momentum of the electron.
[h = 6.63 × 10-34 J∙s, π = 3.142, E1 = -13.6 eV]
Solution :
Data : En = – 0.85 eV, h = 6.63 × 10-34 J∙ s, π = 3.142, E1 = -13.6 eV
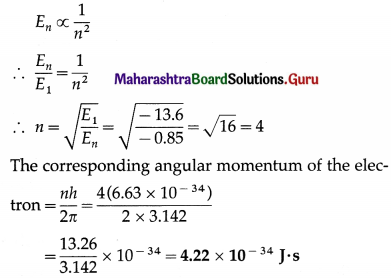
Question 13.
A photon of energy 12.75 eV is absorbed by an electron in the ground state of a hydrogen atom and raises it to an excited state. Find the quantum number of this state.
Solution:
Data : hv = 12.75 eV
The energy of the electron in the ground state of the hydrogen atom, E1 = -13.6 eV
On absorbing a photon of energy hv = 12.75 eV,
its final energy state is
E1 = Ei + hv = – 13.6.+ 12.75 = -0.85 eV
En = \(-\frac{13.6}{n^{2}}\) eV
∴ Ef = -0.85 = \(-\frac{13.6}{n^{2}}\)
∴ n = \(\sqrt{\frac{13.6}{0.85}}\) = 4
∴ The principal quantum number of the final state of the electron = 4
Question 14.
Determine the linear momentum of the electron in the second Bohr orbit in a hydrogen atom. Hence determine the linear momentum in the third Bohr orbit.
Solution:
The linear speed of the electron in the nth Bohr orbit,
vn = \(\frac{e^{2}}{2 \varepsilon_{0} n h}\)
∴ The linear momentum of the electron in the second orbit,
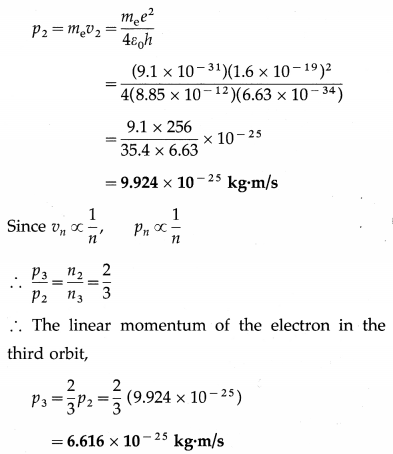
Question 15.
A quantum of monochromatic radiation of wavelength λ is incident on an hydrogen atom that takes it from the ground state to the n = 3 state. Find λ and the frequency of the radiation.
[E1 = -13.6 eV, E3 = -1.51 eV]
Solution :
Data : ni = 3, nf = 1, E1 = -13.6 eV, E3 = 1.51 eV, h = 6.63 × 10-34 J∙s, e = 1.602 × 10-19 C, c = 3 × 108 m/s
The energy of the incident radiation,
hv = E3 – E1
∴ The frequency of the incident radiation,
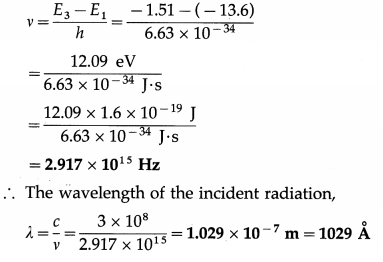

Question 16.
A hydrogen atom undergoes a transition from a state with n = 4 to a state with n = 1. Calculate the change in the angular momentum of the electron and the wavelength of the emitted radiation.
[h = 6.63 × 10-34 J∙s, R = 1.097 × 107 m-1]
Solution :
Data : h = 6.63 × 10-34 J∙s, R = 1.097 × 107 m-1
(i) The angular momentum of the electron in the nth orbit of the hydrogen atom is
Ln = \(\frac{n h}{2 \pi}\)
The change in the angular momentum when the electron jumps from the 4th orbit to the 1st orbit is
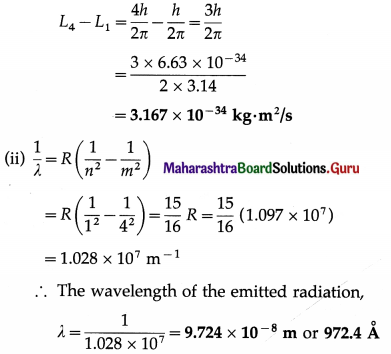
Question 17.
Find the Rydberg constant given the energy of the electron in the second orbit in hydrogen atom is -3.4 eV.
Solution:
Data: E2 = -3.4 eV = -3.4 × 1.6 × 10-19 J, h = 6.63 × 10-34 J∙s, c = 3 × 108 m/s
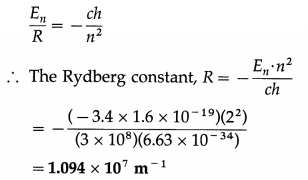
Question 18.
Find the energy of the electron in eV in the third Bohr orbit of the hydrogen atom.
[R = 1.097 × 107 m -1, c = 3 × 108 m/s, h = 6.63 × 10-19 J∙s, e = 1.6 × 10-19 C]
Solution:
Data : n = 3, R = 1.097 × 107 m -1, c = 3 × 108 m/s, h = 6.63 × 10-19 J∙s, e = 1.6 × 10-19 C
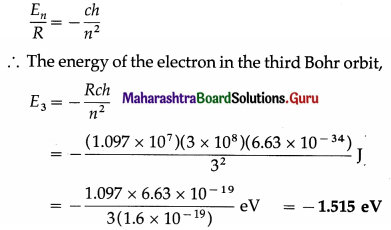
Question 19.
Calculate the wavelength of the first two lines of the Balmer series in the hydrogen spectrum.
Solution :
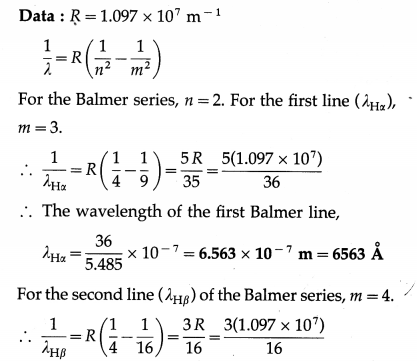
∴ The wavelength of the second Balmer line,
λHβ = \(\frac{16}{3.291}\) × 10-7 = 4.862 × 10-7 m = 4862 Å

Question 20.
The wavelength of H line of the Balmer series is 4860Å. Calculate the wavelength of the Balmer Hx line.
Solution:
Data : λβ = 4860 Å
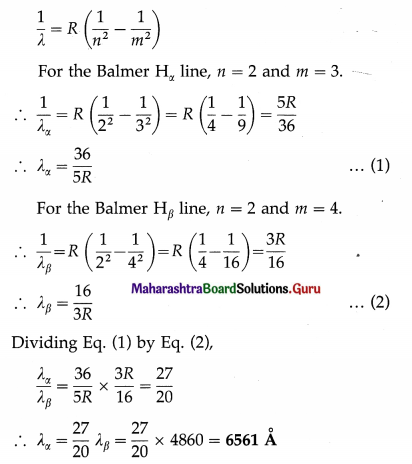
Question 21.
The short wavelength limit of the Lyman series is 911.3 Å. Compute the short wavelength limit of the Balmer series.
Solution:
Data : λ∞L = 911.3 Å
The wavelengths of the lines in the Lyman series are given by
\(\frac{1}{\lambda}=R\left(\frac{1}{1^{2}}-\frac{1}{m^{2}}\right)\) (m = 2, 3, 4, ……..)
For the shortest wavelength line, m = ∞. Therefore, the short wavelength limit of the Lyman series is given by
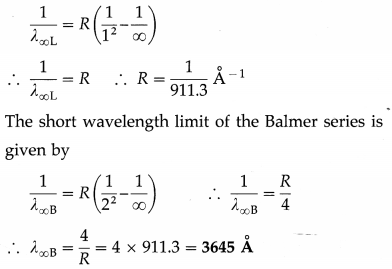
Question 22.
The second member of the Balmer series for the hydrogen atom has wavelength 4860 Å. Calculate Rydberg’s constant.
Solution:
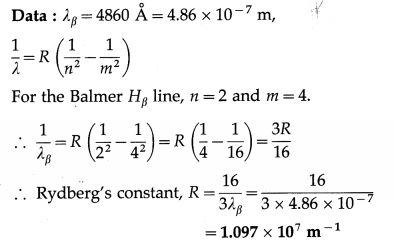
Question 23.
Find the shortest wavelength of the Paschen series, given that the longest wavelength of the Balmer series in the hydrogen spectrum is 6563 Å.
Solution:
Let λBα and λP∞ be the wavelength of the first line, i.e., the longest wavelength line, of the Balmer series and the shortest wavelength of the Paschen series, respectively.
Data : λBα = 6560 Å
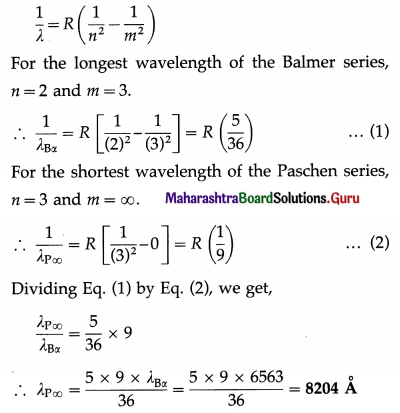
Question 24.
Find the longest wavelength in the Paschen series. [R = 1.097 × 107 m-1]
Solution:
Data: R = 1.097 × 107 m-1
\(\frac{1}{\lambda}=R\left(\frac{1}{n^{2}}-\frac{1}{m^{2}}\right)\)
For the longest wavelength line in the Paschen series, m = 4 and n = 3.
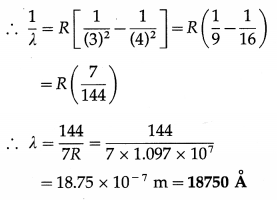

Question 25.
Find the ratio of the longest to shortest wavelengths in the Paschen series.
Solution:
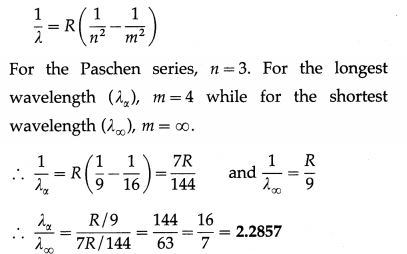
Question 26.
Find the ratio of the longest wavelength in the Paschen series to the shortest wavelength in the Balmer series.
Solution:
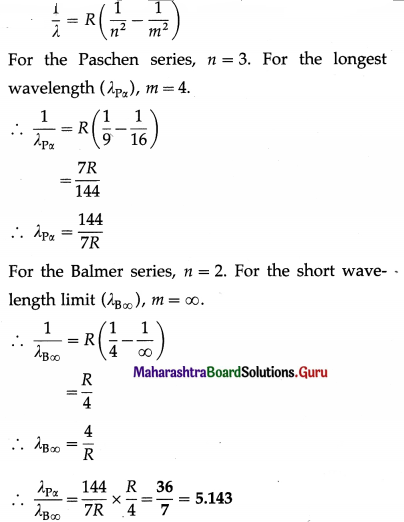
Question 27.
Compute the shortest and the longest wavelength in the Lyman series of hydrogen atom.
Solution:
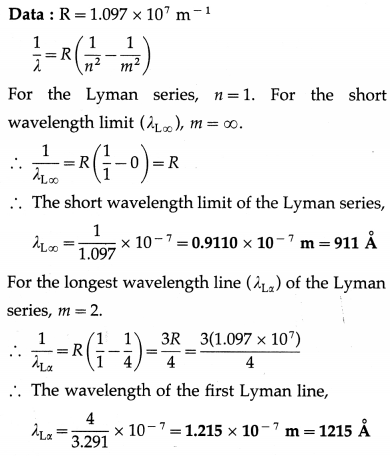

Question 28.
The wavelength of the first line of the Balmer series is 6563 Å. Calculate the wavelength of the first line of (i) the Lyman series (ii) the Paschen series.
Solution:
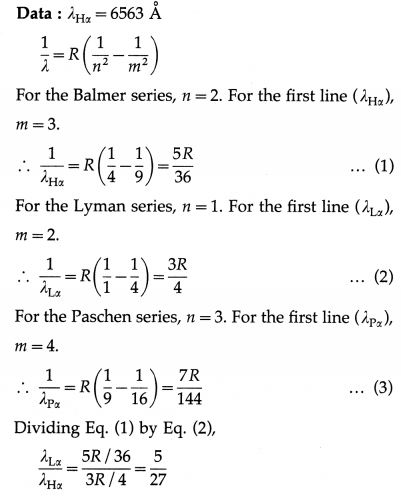
∴ The wavelength of the first line of the Lyman series,
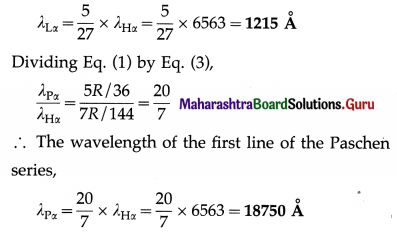
Question 31.
Name the constituents of an atomic nucleus. What is a nucleon?
Answer:
The constituents of an atomic nucleus are (1) the proton, a positively charged particle (2) the neutron, a neutral (uncharged) particle. The term nucleon (nuclear constituent) refers to a proton as well as a neutron.
[Note : The electron was discovered by J. J. Thomson in 1897. The proton was discovered by Ernest Rutherford in 1919. The neutron was discovered in 1932 by James Chadwick (1891-1974), British physicist. The existence of the neutron and the deuteron was predicted by Rutherford in 1920. The proton has a mass about 1836 times that of the electron, but the magnitude of the electric charge is the same for both. The mass of the neutron is slightly more than that of the proton.]
Question 32.
Define (i) atomic number (ii) mass number. Give their symbols.
Answer:
- The number of protons in the nucleus of an atom of an element is called the atomic number of the element. It is also known as the proton number.
It is denoted by Z.
- The number of nucleons (protons and neutrons) in the nucleus of an atom is called the mass number or the atomic mass number. It is denoted by A.
[Notes : (i) The number of neutrons in the nucleus of an atom is known as the neutron number, denoted by N. It is usually greater than Z, with the exceptions of helium (2 protons, 2 neutrons) and hydrogen (1 proton, no neutron) (ii) A = Z + N.]
Question 33.
Write the atomic symbol for an element giving the atomic number and mass number. Give two examples. Which of the two numbers is characteristic of the element? Why?
Answer:
An atom is represented as \(\frac{A}{Z}\)X, where X is the chemical symbol for the element, Z is the atomic number and A is the mass number.
Examples : Fluorine, \(\begin{aligned}
&19 \\
&9
\end{aligned}\)F; Phosphorus, \(\begin{aligned}
&31 \\
&15
\end{aligned}\)P; Gold, \(\begin{aligned}
&197 \\
&79
\end{aligned}\)Au.
The atomic number of an element, which is the number of protons in the nucleus of an atom of the element, is characteristic of the element. It equals the number of electrons in the atom and hence determines the chemical properties of the element and its place in the modern periodic table.

Question 34.
What are isotopes? Give an example.
Answer:
Atoms of an element having the same atomic number (Z) but different mass numbers (A) are called the isotopes of that element.
Isotopes of an element have different neutron numbers but the same chemical properties.
Example : Hydrogen has three isotopes, namely, hydrogen \( (\begin{aligned}
&1 \\
&1
\end{aligned}\) H), deuterium \( (\begin{aligned}
&2 \\
&1
\end{aligned}\) D) and tritium \( (\begin{aligned}
&3 \\
&1
\end{aligned}\) T). Deuterium and tritium have one and two neutrons in their nuclei, respectively, in addition to the single proton (Z = 1).
Question 35.
What are isobars? Give an example.
Answer:
Atoms of different elements that have the same mass number (A) but different atomic numbers (Z) are called isobars.
Although isobars have the same mass number, they are different elements because the chemical nature of an element is determined by its atomic number. Isobars have different neutron numbers.
Example : \(\begin{array}{r}
13 \\
6
\end{array}\)C and \(\begin{array}{r}
13 \\
7
\end{array}\)N are isobars. They have
the same mass number, A (viz., 13), but their different proton numbers, Z (6 and 7) make them different elements.
Question 36.
What are isotones? Give an example.
Answer:
Atoms of different elements that have the same neutron number (N) but different atomic numbers (Z) are called isotones.
Although isotones have the same neutron number, they are different elements because the chemical nature of an element is determined by its atomic number.
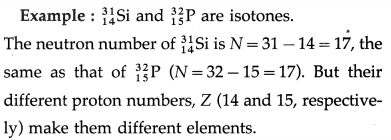
Question 37.
What is unified atomic mass unit? Express it in J/c2 and MeV/c2.
Answer:
The unified atomic mass unit is an accepted but non-SI unit of mass. It is defined to be equal to \(\frac{1}{12}\) of the mass of a free atom of the isotope of carbon with mass number 12 which is at rest and in its ground state. It is denoted by u.
Its value in SI unit is obtained experimentally. 1 u = 1.660538782 (83) × 10-27 kg, with the standard uncertainty in the last two digits given in v parenthesis.
Taking, 1 u = 1.660538782 × 10-27 kg
c = 2.99792458 × 108 m/s,
e = 1.602176462 × 10-19 C and
using the relation E = me2 { ≡ m ≡ E / c2),
we get, 1 u = 1.49241783 × 10-10 J/c2
≅ 1.492 × 10-10 J/c2
and 1 u = 931.494042 MeV / c2
≅ 931.5 MeV/c2

Question 38.
How is the nuclear size determined ? State the relation between nuclear size (radius) and mass number.
Answer:
The nuclear size is determined from particle scattering experiments using fast electrons or neutrons. The de Broglie wavelength of the bombarding electrons or neutrons should be less than the radius of the nucleus under study.
It is found that the volume of a nucleus is directly proportional to the mass number A, i.e., to the number of nucleons in the nucleus. For many purposes, nuclei may be assumed to be spherical.
Thus, a nucleus of radius R has a volume \(\frac{4}{3}\) πR3
∴ R3 ∝ A or R ∝ A\(\frac{1}{3}\)
∴ R = R0A\(\frac{1}{3}\)
where R0 ≈ 1.2 × 10-15 m = 1.2 fm.
∴ R ≈ 1.2 AA\(\frac{1}{3}\) fm
[Note : A nucleus does not have a sharp boundary. Also, electron scattering and neutron scattering yield slightly different values of R0. Hence, the relation above is only representative of effective nuclear size. 1 femtometre or 1 fm = 10-15 m; an earlier non-SI unit of the same value called fermi, in honour of Enrico Fermi (1901-54), Italian-US nuclear physicist, is no longer accepted in SI.]
Question 39.
Nuclear density is essentially the same for all nuclei. Justify.
Given 1 u = 1.66 × 10-27 kg and R0 ≈ 1.2 fm, estimate the nuclear density.
Answer:
Relative atomic mass rounded to the nearest integral value equals the atomic mass number A. Thus, ignoring the masses of the atomic electrons and binding energies, nuclear mass expressed in unified atomic mass unit = A u. Also, it is experimentally found that the volume of a nucleus is directly proportional to the mass number A. As both nuclear mass and volume are proportional to the mass number, nuclear density is essentially the same for all nuclei.
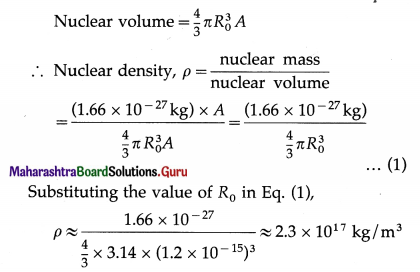
The order of nuclear density is 1017 kg/m3.
[Note : Some stars, with masses between 1.4\(M_{\odot}\) and 3\(M_{\odot}\), where \(M_{\odot}\) denotes the mass of our Sun, undergo supernova at the end of their active life and collapse into neutron stars of densities comparable with nuclear v density.]
Question 40.
Define mass defect and state an expression for it.
Answer:
The difference between the sum of the masses of all the individual nucleons in a nucleus and the mass of the nucleus is known as the mass defect.
Mass defect, ∆m = (Zmp + Nmn) – M where mp is the proton mass, mn is the neutron mass, M is the mass of the nucleus, Z is the atomic number and N = A – Z is the neutron number.

Question 41.
Explain the term nuclear binding energy and express it in terms of mass defect. What is binding energy per nucleon? Write the expression for it.
Answer:
In the atomic nucleus, the protons and the neutrons are bound together by a strong, short range and charge independent, attractive force called the nuclear force. It is necessary to supply energy to break the nucleus. The minimum energy required to separate a nucleus into its free constituents, i.e., protons and neutrons, is known as the nuclear binding energy. It is the mass energy of the nucleons minus the mass energy of the nucleus.
The mass defect of a nucleus, of mass M, mass number A, proton number Z and neutron number N = A – Z, is
∆m = (Zmp + Nmn) – M
where, mp is the proton mass and mn is the neutron mass. Then, from Einstein’s mass-energy relation,
nuclear binding energy = ∆mc2
= [(Zmp -(- Nmn) – M] c2 (in joule)
= [(Zmp + Nmn) – M] \(\frac{c^{2}}{e}\) (in eV)
where c is the speed of light in free space and e is the elementary charge. In calculations using the above equations, mp is replaced by mH (mass of hydrogen atom). M is taken to be the atomic mass and not nuclear mass because the electron masses cancel out and the difference in electronic binding energies can be ignored as these are 106 times smaller than the nuclear binding energy.
∴ Nuclear binding energy
≅ [(ZmH + Nmn) – Matom] c2 (in joule)
The minimum energy required on the average to separate a nucleon from a given nucleus is called the binding energy per nucleon for that nucleus. It is the nuclear binding energy for a nucleus divided by its mass number.
∴ Binding energy per nucleon = \(\frac{\Delta m c^{2}}{A}\)
= [(Zmp + Nmn) – M] \(\frac{c^{2}}{A}\)
≅ [(ZmH + Nmn) – Matom] \(\frac{c^{2}}{A}\)
Question 42.
What is the significance of binding energy per nucleon?
Answer:
The greater the binding energy per nucleon in a nucleus, the greater is the minimum energy needed to remove a nucleon from the nucleus. Thus, binding energy per nucleon indicates the stability of a nucleus.
[Note : The binding energy per nucleon is high when both Z and N are even numbers, and such nuclei are most common. Nuclei with both Z and N odd are very rare.]
Question 43.
What are stable nuclei? What decides nuclear stability? What are the properties of nuclear force?
Answer:
Those nuclei which for certain combinations of neutrons and protons do not spontaneously disintegrate are called stable nuclei. There are two aspects that decide the stability of a nucleus. Firstly, the existence of nuclear energy levels implies certain configurations to achieve potential energy minimum, and secondly, the balance of forces.
Just like energy levels in atoms, nuclear energy levels are filled in sequence obeying the exclusion principle. Thus, there is a tendency for N to equal Z, or to have both even Z and even N.
Properties of the nuclear force :
(1) The nucleons in a nucleus are held .together by the attractive strong nuclear force. This force is much stronger than gravitational force and electromagnetic force.
(2) Nucleons interact strongly only with their nearest neighbours because the nuclear force has an extremely short range. Gravitational force and electromagnetic force are long range forces. They tend to zero only when the separation between two particles tends to infinity.
(3) Inside a nucleus, this force appears to be the same between two protons, a proton and a neutron, and two neutrons. However, between two protons there is also Coulomb repulsion which has a much longer range and, therefore, has appreciable magnitude throughout the entire nucleus. In nuclei having 2 ≤ Z ≤ 83, with neutrons present, the nuclear force is strong enough to overcome the Coulomb repulsion.
For light nuclei (A < 20), N ≥ Z, but is never smaller (except in \(\begin{aligned} &1 \\ &1 \end{aligned}\)H and \(\begin{aligned} &3 \\ &2 \end{aligned}\)H). However, with more than about 10 protons, an excess of neutrons is required to form a stable nucleus; for high atomic numbers, N/Z = 1.6. For Z > 83, even an excess of neutrons cannot prevent spontaneous disintegration and there are no stable nuclei.
[Note : The strength of the nuclear force is evident from the nuclear binding energy.]
Question 44.
Draw a neat labelled graph showing the variation of binding energy per nucleon as a function of mass number. What can we infer from the
graph?
Answer:
Figure shows the plot of the binding energy per nucleon (BE/A, in MeV per nucleon) against mass number A.
From Figure, we can draw the following inferences :
(1) The greater the binding energy per nucleon, the more stable is the nucleus because greater is the minimum energy needed to remove a nucleon. Thus, the nuclei appearing high on the plot are more tightly bound.
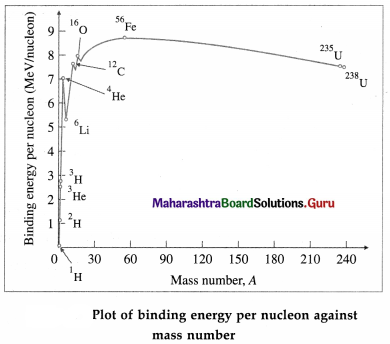
(2) BE/A has a maximum value of about 8.8 MeV per nucleon at A = 56 (56Fe nuclide) and then decreases to 7.6 MeV per nucleon at A = 238 (238U nuclide). Thus, the iron nuclide 56Fe has the maximum binding energy per nucleon and is the most stable nuclide. Also, the peak at A = 4 shows that the 4He nucleus (α particle) has higher binding energy per nucleon compared to its neighbours in the periodic table and is exceptionally stable.
(3) The increase in BE /A as A decreases from 240 to 60 shows that if a heavy nucleus splits into two medium-sized fragments, each of the new nuclei will have more BE / A than the original nucleus. The binding energy difference, which can be very large, will then be released. The process of splitting a heavy nucleus is called nuclear fission. The energy released in a fission of 235U nucleus is about 200 MeV.
(4) Joining together, or fusing, two very light nuclei to form a single nucleus will also lead to larger BE/A in the new heavier nucleus. Again, the binding energy difference will be released. This process,which is called nuclear fusion, is also a very effective way of obtaining energy.

45. Solve the following :
Question 1.
Find the nuclear radii of 206Pb and 208Pb.
Solution:
Data : R0 = 1.2 × 10-15 m
Nuclear radius, R=R0A\(\frac{1}{3}\)
(i) For 206Pb, A = 206
∴ R = (1.2 × 10-15) (206)\(\frac{1}{3}\)
= 1.2 × 10-15 × 5.906
= 7.087 × 10-15 m = 7.087 fm
(ii) For 208 Pb, A = 208
∴ R = (1.2 × 10-15)(208)\(\frac{1}{3}\)
= 1.2 × 10-15 × 5.925
= 7.11 × 10-15 m = 7.11 fm
Question 2.
Given the atomic mass of the isotope of iron 56Fe is 55.93 u, find its nuclear density.
Solution :
Data : A = 56, m = 55.93 u = 55.93 × 1.66 × 10-27 kg, R0 = 1.2 × 10-15 m .
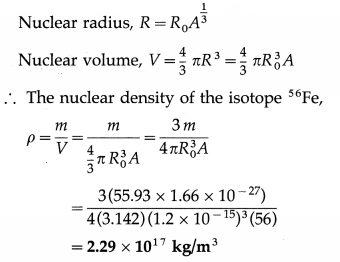
Question 3.
Given the nuclear radius of 16O is 3.024 fm, find that of 235U.
Solution:
Data : A1 = 16 and R1 = 3.024 fm (for 16O),
A2 = 235 (for 235U)
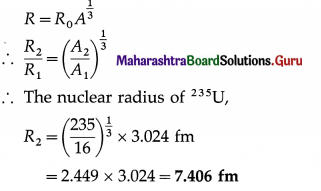
Question 4.
The mass defect of He nucleus is 0.0304 u. Calculate its binding energy.
Solution:
Data: ∆m = 0.0304 u, 1 u = 931.5 \(\frac{\mathrm{MeV}}{c^{2}}\)
The binding energy of He nucleus
= ∆mc2
= 0.0304 × 931.5
= 28.32 MeV
Question 5.
Calculate the mass defect and binding energy of \(\begin{aligned}
&59 \\
&27
\end{aligned}\) Co which has a nucleus of mass 58.933 u. [Take mp = 1.0078 u, mn = 1.0087 u]
Solution:
Data: mp = 1.0078 u, mn = 1.0087 u, mCo = 58.933 u,
1 u = 931.5 \(\frac{\mathrm{MeV}}{c^{2}}\)
For \(\begin{aligned}
&59 \\
&27
\end{aligned}\)Co, A = 59, Z = 27
∴ N = A – Z = 59 – 27 = 32
The mass defect,
∆m=(Zmp + Nn) – mCo
= (27 × 1.0078 + 32 × 1.0087) – 58.933
= (27.2106 + 32.2784) – 58.933
= 59.4890 – 58.933 = 0.556 u
∴ The binding energy
= ∆mc2
= 0.556 uc2 × 931.5 \(\frac{\mathrm{MeV}}{uc^{2}}\) = 517.8 MeV

Question 6.
Find the mass energy of a particle of mass 1 u in joule and electronvolt.
Solution:
Data : m = 1 u = 1.66 × 10-27 kg, c = 3 × 108 m/s,
1 eV = 1.602 × 10-19 J
The mass energy,
E = mc2
= (1.66 × 10-27) (3 × 108)8
= 1.66 × 9 × 10-11 n
= 1.494 × 10-10 J
= \(\frac{1.494 \times 10^{-10}}{1.602 \times 10^{-19}}\) eV
= 9.326 × 108 eV or 932.6 MeV
[Note : The answer differs from the accepted value of about 931.5 MeV because of the rounding off errors in the data used.]
Question 7.
Find the mass energy of a proton at rest in MeV. [mp = 1.673 × 10-27kg]
Solution:
Data : mp = 1.673 × 10-27 kg, c = 3 × 108 m/s,
1 eV = 1.602 × 10-19 J
∴ 1 MeV = 106 × 1.602 × 10-19 J = 1.602 × 10-13 J
The mass energy of a proton at rest,
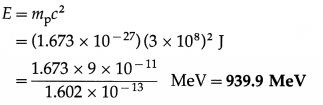
Question 8.
Find the mass energy of a particle of mass 1 g in joule.
Solution:
Data : m = 1 g = 1 × 10-3 kg, c = 3 × 108 m/s,
1 eV = 1.602 × 10-19 J
The mass energy,
E = mc2
= (1 × 10-3)(3 × 108)2
= 9 × 1013 J
Question 46.
What is radioactivity? OR Define radioactivity.
Answer:
Radioactivity is the phenomenon in which unstable nuclei of an element spontaneously distintegrate into nuclei of another element by emitting a or β particles accompanied by γ-rays. Such transformation is known as radioactive transformation or radioactive decay.
Question 47.
Who discovered radioactivity? Give a brief account of the first observation of radioactivity.
Answer:
Antoine Henri Becquerel (1852-1908), French physicist, discovered radioactivity in 1896.
He had kept photographic plates wrapped in a thick black paper in a drawer of his desk. Later, he also kept uranium salts near the photographic plates. After some days he developed the photographic plates and was surprised to find that they were fogged although he had protected them from light. Becquerel concluded that uranium salts must be emitting some invisible rays which affected the photographic plates. In this way, radioactivity was discovered by Becquerel.

Question 48.
What is a radioactive element? Give two examples of radioactive elements.
Answer:
The element which exhibits the property of radioactivity, i.e., spontaneous disintegration of unstable nuclei of the element by emission of α or β particles accompanied by γ-rays is called a radioactive element. Examples : Uranium, thorium, polonium, radium, actinium.
Question 49.
Name the three radioactive decay processes. State the nature of particle/radiation emitted in each process. What is meant by the Q value or Q factor of the decay?
Answer:
The processes by which a radioactive element can decay are :
(1) α-decay, by emission of an α-particle (\(\begin{aligned}
&4 \\
&2
\end{aligned}\) He nucleus),
(2) β-decay, by emission of an electron (\(\begin{aligned}
&0 \\
&-1
\end{aligned}\)e) or a positron \(\begin{aligned}
&0 \\
&1
\end{aligned}\)e), and
(3) γ-decay, by emitting electromagnetic radiations (γ-rays) of very short wavelength of about 10-12 m to 10-14 m.
In a radioactive decay, the difference in the energy equivalent of the mass of the parent atom and that of the sum of the masses of the products is called the Q value or Q factor of the decay. It is also called disintegration energy.
Question 50.
State the observations which lead to the conclusion that radioactivity is a nuclear phenomenon.
Answer:
The rate of disintegration of a radioactive material is not affected by changes in physical and chemical conditions such as (1) temperature and pressure (2) action of electric and magnetic fields (3) chemical composition of the material. The above changes affect the orbital electrons, but not the nucleus. Therefore, we conclude that radioactivity is a nuclear phenomenon.
Question 51.
State the nature and properties of a-particles.
Answer:
Nature of α-particles :
(1) An alpha particle is a helium nucleus, i.e., a doubly ionized helium atom. It consists of two protons and two neutrons.
(2) Mass of the α-particle ≅ 4u
Charge on the α-particle = 2 × charge on the proton
Properties of α-particles :
(1) Of the three types of radioactive radiations, α- particles have the maximum ionizing power. It is about 100 times that of β-particles and 104 times that of γ-rays.
(2) They have the least penetrating power, about 100 times less than that of β-particles and 104 times less than that of γ-rays. They can pass through very thin sheets of paper but are scattered by metal foils and mica. Since α-particles produce intense ionization in a medium, they lose their kinetic energy quickly. As a result, they do not penetrate more than a few centimetres (about 2.7 cm to 8.6 cm) in air under normal conditions.
(3) They are deflected by electric and magnetic fields since they are charged particles. Their deflection is less than that of β-particles in the same field.
(4) They affect photographic plates.
(5) They cause fluorescence in fluorescent materials such as zinc sulphide.
(6) They emerge from the nuclei with tremendous speeds in the range of \(\frac{1}{100}\)th to \(\frac{1}{10}\)th of the speed of light in free space.
(7) They destroy living cells.
[Note : α-rays and β-rays were discovered by Henri BecquereL]

Question 52.
State the nature and properties of β-particles.
Answer:
Nature of β-particles : A β-particle is an electron or a positron.
Properties of β-particles :
- β-particles have a moderate ionizing power. It is about 100 times less than that of α-particles, but 100 times more than that of γ-rays.
- They have a moderate penetrating power. It is about 100 times more than that of α-particles, but 100 times less than that of γ-rays.
- They are deflected by electric and magnetic fields. Their deflection is more than the deflection of α-particles in the same field but in the opposite direction.
- They affect photographic plates.
- They cause fluorescence in a fluorescent material such as zinc sulphide.
- Their energies and speeds are very high. Their speed is of the order of 108 m/s. Some β-particles have speeds of the order of 0.99 c, where c is the speed of light in free space.
- They cause more biological damage than α-particles, because they have more penetrating power.
[Note : When a nucleus emits an electron, one of its neutrons changes to a proton; the electron is accompanied by a neutral and almost massless particle called antineutrino \(\bar{v}_{\mathrm{e}}\) with which the electron shares its energy and momentum. Hence, β-particles are emitted with speeds ranging from about 0 to 0.99 c.
The properties of a positron are identical to those of an electron except that it carries a positive charge of the same magnitude as an electron. A positron emission in a β+ decay is accompanied by a neutrino ve. A positron is an anti-particle of an electron, and an antineutrino is an antiparticle of neutrino.
The existence of a small neutral particle, emitted simultaneously with the electron in β–-decay, was proposed in 1931 by Wolfgang Pauli (1900 -1958), Austrian-US theoretical physicist. It was confirmed experimentally in 1956 by Frederic Reines and Clyde Lorrain Cowan, Jr., US physicists. This neutral particle, that appears in β+ -decay, was called the neu-trino. It travels with a speed very close to that of light in free space. The existence of positron (in 1928) and other antiparticles was predicted by Paul Adrien Maurice Dirac (1902 – 84), British theoretical physicist. All these predictions were eventually confirmed experimentally; the positron was discovered in 1932 by Carl David Anderson, US physicist.]
Question 53.
State the nature and properties of γ-rays.
Answer:
Nature of γ-rays :
- γ-rays are electromagnetic waves of very short wavelength (about 10-12 m to 10-14 m).
- They are uncharged.
Properties of γ-rays :
- γ-rays produce feeble ionization. Their ionizing power is 104 times less than that of a-particles and 100 times less than that of β-particles.
- They have the maximum penetrating power. It is about 100 times that of β-particles and 104 times that of α-particles.
- They are not deflected by electric and magnetic fields as they are uncharged.
- They affect photographic plates.
- They cause fluorescence in a fluorescent material such as zinc sulphide.
- Their speed in free space is 3 × 108 m/s( the same as that of light waves and X-rays in free space).
- γ-rays can be diffracted by crystals. In recent times, γ-ray diffraction has emerged as a powerful tool in structural and defect studies of crystals.
- They destroy living cells and tissues and are used for destroying cancer cells.
[Note : γ-rays (not established clearly in Berquerel’s work) were discovered in 1900 by Paul Villard (1860-1934), French physicist.]
Question 54.
(a) What is α-decay? What is the consequence of an α-decay on a radioactive element? What is the Q value or Q factor in this case ?
Q = [mU – mTh – mα]c2
(b) What is β-decay ? What is the consequence of a β-decay on a radioactive element? What is the Q value or Q factor in this case ?
Answer:
(a) A radioactive transformation in which an a-particle is emitted is called α-decay.
In an α-decay, the atomic number of the nucleus decreases by 2 and the mass number decreases by 4.
Example : \({ }_{92}^{38} \mathrm{U} \rightarrow{ }_{90}^{234} \mathrm{Th}+{ }_{2}^{4} \alpha\)
Q = [mU – mTh – mα]c2
(b) A radioactive transformation in which a β-particle is emitted is called β-decay.
In a β -decay, the atomic number of the nucleus increases by 1 and the mass number remains unchanged.
Example : \({ }_{90}^{23} \mathrm{Th} \rightarrow{ }_{91}^{234} \mathrm{~Pa}+{ }_{-1}^{0} e+\bar{v}_{\mathrm{e}}\)
where \(\bar{v}_{\mathrm{e}}\) is the antineutrino emitted to conserve the momentum, energy and spin.
Q = [mTh – mPa – me]c2
In a β+-decay, the atomic number of the nucleus decreases by 1 and the mass number remains unchanged.
Example : \({ }_{15}^{30} \mathrm{P} \rightarrow{ }_{14}^{30} \mathrm{Si}+{ }_{+1}^{0} e+v_{\mathrm{e}}\)
where ve is the neutrino emitted to conserve the momentum, energy and spin.
Q = [mP – mSi – me]c2
[Note : The term fi particle refers to the electron (or positron) emitted by a nucleus.]

Question 55.
Why are α- and β-particle emissions often accompanied by γ-rays?
Answer:
A given nucleus does not emit α- and βparticles simultaneously. However, on emission of α or β-particles, most nuclei are left in an excited state. A nucleus in an excited state emits a γ-ray photon in a transition to the lower energy state. Hence, α- and β-particle emissions are often accompanied by γ-rays.
Question 56.
State the law of radioactive decay and express it in the exponential form.
OR
State the law of radioactive decay. Hence derive the relation N = N0e-λt, where the symbols have their usual meanings.
OR
Show that the number of nuclei of a radioactive material decreases exponentially with time.
Answer:
Law of radioactive decay : At any instant, the rate of radioactive distintegration is directly proportional to the number of nuclei of the radioactive element present at that instant.
Derivation : Let N0 be the number of nuclei present at time t = 0, and N the number of nuclei present at time t.
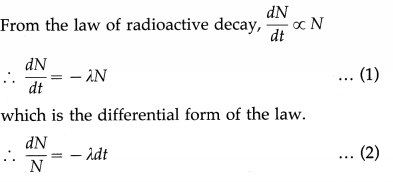
where λ is a constant of proportionality called the radioactive decay constant or the distintegration constant. It is a constant for a particular radioactive element. The minus sign indicates that N decreases as t increases.
Integrating Eqn (1),
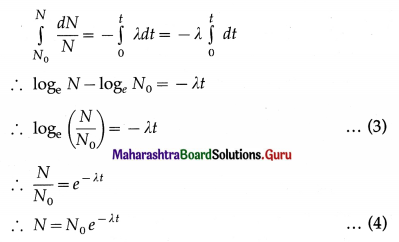
This is the exponential form of the law of radioactive decay. It shows that the number of nuclei present decreases exponentially with time.
[Note : This equation is also written in the form
N(t) = N0e-λt]
Question 57.
If the number of nuclei of a radioactive substance becomes \(\frac{1}{e}\) times the initial number in 10 days, what is the decay constant of the substance ?
Answer:
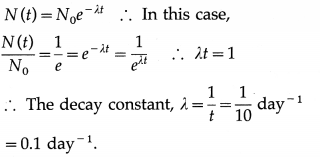

Question 58.
What is meant by the activity of a sample of radioactive element? State the expression for it and also the units.
Answer:
The rate of disintegration of a sample of a radioactive element is called its activity. Let A denote the activity at time t and A0 the initial activity. Then,
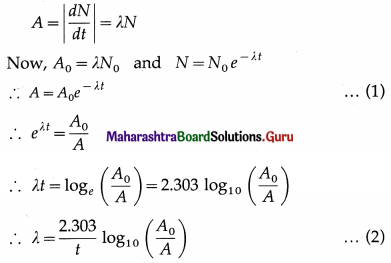
The SI unit of activity is called the becquerel (Bq) in honour of Henri Becquerel. 1 Bq = 1 disintegration per second.
The earlier unit, the curie (Ci) was based on the activity of 1 gram of 226Ra. 1 Ci = 3.7 × 1010 Bqn It was named after Marie Curie, Polish-bom French chemist.
[Note : Eqn (1) is also written in the form A (t) = A0e-λt]
Question 59.
Define half-life a radioactive element and obtain the relation between half-life and decay constant.
Answer:
The half-life of a radioactive element is defined as the average time interval during which half of the initial number of nuclei of the element disintegrate.
Let N0 be the number of nuclei of a radioactive element present at time t = 0 and N, the number of nuclei present at time t. From the law of radioactive decay,
N = N0e-λt
where λ is the decay constant of the element.
If T is the half-life of the element, then, N = \(\frac{N_{0}}{2}\) when t = T.
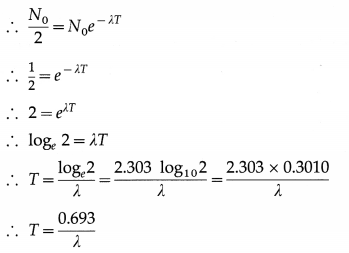
This is the relation between the half-life and the decay constant of a radioactive element.
[Note : Radioactive decay law is statistical in nature and applicable only when the number of nuclei in the sample under consideration is very large. λ gives the probability that a nucleus of the element will decay in one second. We cannot know the exact number of nuclei that would decay in a given time interval. Hence, the use of the term average in the definition of half-time.]
Question 60.
What is meant by average life or mean life of a radioactive species ? How is it related to the half-life?
Answer:
Let N0 = number of nuclei present at time t = 0 and λ = decay constant of a radioactive species.
| dN | = | λNdt |. ∴ The number of nuclei decaying between time tand t + dt is λN0e-λtdt. The life time of these nuclei is t. The average life or mean life of a radioactive species is denoted by t and is, by definition,
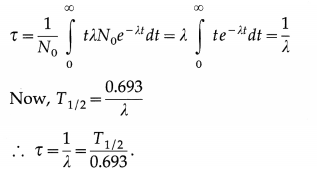
Question 61.
Define decay constant or distintegration constant of a radioactive element. If λ is the decay constant of a radioactive element, show that about 37% of the original nuclei remains undecayed after a time interval of λ-1.
Answer:
The decay constant or disintegration constant of a radioactive element is defined as the ratio of the disintegration rate at an instant to the number of undecayed nuclei of the element present at that instant.
Let N0 be the number of nuclei of a radioactive element present at time t = 0 and N, the number of undecayed nuclei at time t. From the radioactive law,
N = N0e-λt
where λ is the decay constant. At t = λ-1, the fraction of undecayed nuclei is
\(\frac{N}{N_{0}}\) = e-λ × λ-1 = e-1 = \(\frac{1}{e}\)
Since e ≅ 2.718,
\(\frac{N}{N_{0}}=\frac{1}{2.718}\) = 0.3679
Therefore, about 36.79% ≈ 37% of the original nuclei remains undecayed after a time λ-1. Since λ is the probability that a nucleus of the element will decay in one second, λ-1 gives the mean-life or the mean life time τ of the radioactive element measured in second; τ = λ-1.

Question 62.
Show graphically how the number of nuclei (N) of a radioactive element varies with time (t).
Answer:
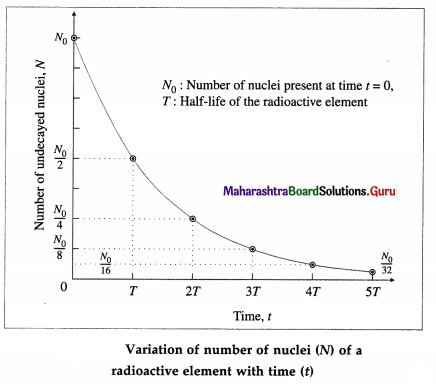
Question 63.
The half-life of a radioactive material is 4 days. Find the time required for 1/4 of the initial number of radioactive nuclei of the element to remain undisintegrated.
Answer:
For t = nT, N = N0/ 2n. In this case, n = 2.
∴ f = 2T = 2 × 4 = 8 days is the required time.
Question 64.
A radioactive sample with half-life 2 days has initial activity 32 μCi. What will be its activity after 8 days?
Answer:
Here, t = 8d and T = 2d
∴ t =4T
For t = nT, N = N0/ 2n and activity ∝ N
∴ A = A0/24 = A0/16 = 32 /16
= 2 μCi is the required activity.
Question 65.
In successive radioactive decay if the decrease in mass number is 32 and the decrease in atomic number is 8, how many (i) α particles (ii) β particles are emitted in the process ?
Answer:
- Number of α-particles emitted = 32/4 = 8
- Number of β-particles emitted = 16 – 8 = 8.
66. Solve the following :
Question 1.
The decay constant of a radioactive substance is 4.33 × 10-4 per year. Calculate its half-life and average life.
Solution:
Data : λ = 4.33 × 10-4 per year
The half-life period of the radioactive substance.
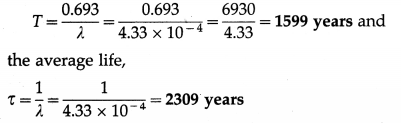
Question 2.
The half-life of \(\begin{array}{r}
226 \\
88
\end{array}\)Ra is 1620 y. Find its decay constant in SI unit.
Solution:
Data: T = 1620 y = 1620 × 365 × 8.64 × 104 s
= 5.109 × 1010 s
The decay constant of \(\begin{array}{r}
226 \\
88
\end{array}\)Ra is
λ = \(\frac{0.693}{T}=\frac{0.693}{5.109 \times 10^{10}}\) = 1.356 × 10-11 s-1
Question 3.
The half-life of \(\begin{array}{r}
210 \\
84
\end{array}\)Po is 138 d. Find the time required for 75% of the initial number of radio active nuclei of \(\begin{array}{r}
210 \\
84
\end{array}\)Po to disintegrate.
Solution:
Data: T = 138 d, = \(\frac{N}{N_{0}}=\frac{1}{4}\) (since only 25% of the
initial number of nuclei remains undisintegrated)
In one half-life (t = T), \(\frac{N}{N_{0}}=\frac{1}{2}\). In two half-lives (t = 2T), \(\frac{N}{N_{0}}=\frac{1}{4}\)
∴ The time for 75% of \(\begin{array}{r}
210 \\
84
\end{array}\)Po nuclei to disintegrate is 2T = 2 × 138 = 276 d.
Question 4.
Protactinium \(\begin{array}{r}
233 \\
91
\end{array}\)Pa decays to \(\frac{1}{5}\)th of its initial quantity in 62.7 days. Calculate its decay constant, mean-life and half-life.
Solution:
Data : \(\frac{N}{N_{0}}=\frac{1}{5}\), t = 62.7d
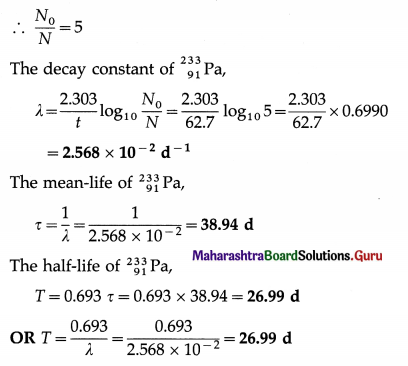
Question 5.
The half-life of \(\begin{array}{r}
210 \\
82
\end{array}\)Pb is 2.2.3 y. How long will it take for its activity to reduce to 30% of the initial activity?
Solution:
Data : T = 22.3 y, A = 0.3 A0
By the radioactive decay law,
N = N0e-λt
∴ λN = λN0e-λt
∴ λ = A0e-λt
where A0 = AN0 is the initial activity and A = λN is the activity at time t.
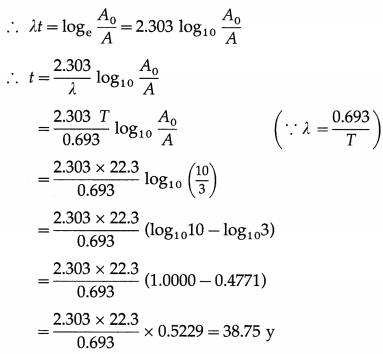
∴It will take 38.75 y for the activity of \(\begin{array}{r}
210 \\
82
\end{array}\)Pb to reduce to 30% of the initial activity.

Question 6.
Radioactive sodium \(\begin{aligned}
&24 \\
&11
\end{aligned}\)Na has half-life of 15 h. Find its decay constant and mean-life. How much of 10 g of \(\begin{aligned}
&24 \\
&11
\end{aligned}\)Na will be left after 24 h?
Answer:
Data : T = 15 h, m0 = 10 g, t = 24 h
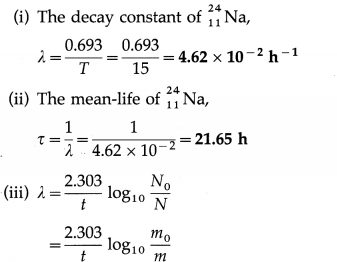
since mass of a sample is directly proportional to the number of atoms or nuclei present.
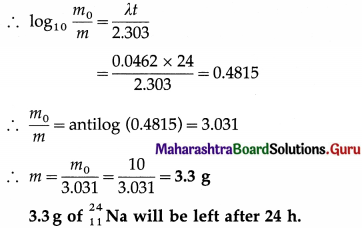
Question 7.
Thorium \(\begin{gathered}
232 \\
90
\end{gathered}\)Th disintegrates into lead \(\begin{gathered}
200 \\
82
\end{gathered}\)Pb. Find the number of α and β particles emitted in the disintegration.
Solution:
An α-particle is a helium nucleus with mass number 4 and atomic number 2. In an α-decay, the mass number of the disintegrating nucleus decreases by 4 and its atomic number decreases by 2.
A β–-particle is an electron with mass number 0 and atomic number – 1. In a β–-decay, the mass number of the disintegrating nucleus remains unchanged and its atomic number increases by 1.
Let x α-particles and y ß-particles be emitted in the disintegration of \(\begin{gathered}
232 \\
90
\end{gathered}\)Th into \(\begin{gathered}
200 \\
82
\end{gathered}\)Pb.
\({ }_{90}^{232} \mathrm{Th} \stackrel{x \alpha+y \beta^{-}}{\longrightarrow}{ }_{82}^{200} \mathrm{~Pb}\)
∴ 232 – 4x – 0(y) = 200
∴ 4x = 232 – 200 = 32
∴ x = 8
Also, 90 – 2x + 1(y) = 82
∴ 90 – 2(8) + y = 82
∴ y = 82 + 16 – 90 = 8
∴ 8 α-particles and 8 ß–-particles are emitted in the decay series of 232Th to 200Pb.
Question 67.
What is nuclear energy?
Answer:
Energy released in a nuclear reaction such as a spontaneous or induced nuclear fission, or nuclear fusion, or in interaction of two nuclei, is called nuclear energy.
[Note : It is far greater from that released in a chemical reaction.]
Question 68.
What is a nuclear reaction? Give one example.
Answer:
A reaction between the nucleus of an atom and a bombarding particle leading to the production of a new nucleus and, in general, the ejection of one or more particles is known as a nuclear reaction.
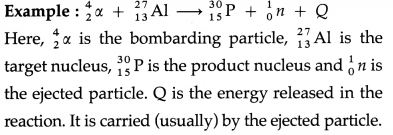
Question 69.
What are the quantities conserved in a nuclear reaction?
Answer:
The total momentum, energy, spin, charge and number of nucleons are conserved in a nuclear reaction.

Question 70.
What is fission? Who discovered nuclear fission?
Answer:
Nuclear fission is a nuclear reaction in which a heavy nucleus of an atom, such as that of uranium, splits into two or more fragments of comparable size, either spontaneously or as a result of bombardment of a neutron on the nucleus (induced fission). It is followed by emission of two or three neutrons.
The mass of the original nucleus is more than the sum of the masses of the fragments. This mass difference is released as energy, which can be enormous as in the fission of 235U.
Nuclear fission was discovered by Lise Meitner, Otto Frisch, Otto Hahn and Fritz Strassmann in 1938.
[Notes : (1) Lise Meitner (1878-1968), Austrian- Swedish physicist and radiochemist. Otto Frisch (1904-79), Austrian-British physicist and Meitner’s nephew. Otto Hahn (1879-1968), German radiochemist. Friedrich Wilhelm “Fritz” Strassman (1902-1980), German chemist. (2) In 1934, Enrico Fermi bombarded uranium with neutrons to produce nuclear reactions leading to formation of transuranic elements (Z > 92). In the process, he had carried out nuclear fission, but he misinterpreted the results. The experiments and analysis carried out by Meitner, Hahn and Strassman, and the theoretical work by Meitner and Frisch led to the discovery of fission in 1938-39.
Frisch named the phenomenon fission. Fermi, for his work in the area of nuclear science, was awarded the 1938 Nobel Prize for physics. Hahn was awarded the 1944 Nobel Prize for chemistry; it was not shared by Meitner as her important role in the discovery of fission came to light much later. (3) The most abundant urnanium isotope 238U (abundance 99.28%) can be fissioned by neutrons with high kinetic energy (called fast neutrons), at least 1.3 MeV. 235U (abundance 0.72%) can be fissioned by thermal (or low energy) neutrons having kinetic energy about 0.025 eV. Some types of nuclear reactors require the natural uranium to be enriched to increase its 235U content to about 3%.]
Question 71.
What are the products of the fission of uranium 235 by thermal neutrons?
Answer:
The products of the fission of 235U by thermal neutrons are not unique. A variety of fission fragments are produced with mass number A ranging from about 72 to about 138, subject to the conservation of mass-energy, momentum, number of protons (Z) and number of neutrons (N). A few typical fission equations are
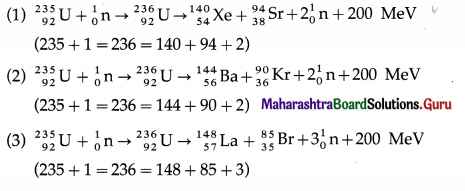
Question 72.
What is nuclear fusion? Give one example with an equation.
Answer:
A type of nuclear reaction in which lighter atomic nuclei (of low atomic number) fuse to form a heavier nucleus (of higher atomic number) with the’ release of enormous amount of energy is called nuclear fusion.
Very high temperatures, of about 107 K to 108 K, are required to carry out nuclear fusion. Hence, such a reaction is also called a thermonuclear reaction.
Example : The D-T reaction, being used in experimental fusion reactors, fuses a deuteron and a triton nuclei at temperatures of about 108 K.
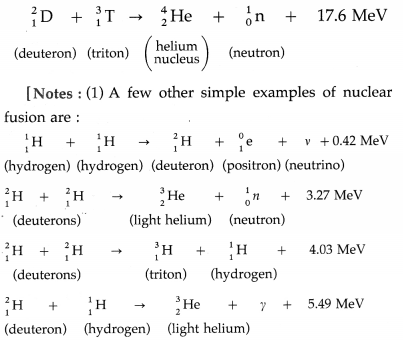
(2) The value of the energy released in the fusion of two deuteron nuclei and the temperature at which the reaction occurs mentioned in the textbook are probably misprints.]

Question 73.
Give one area of application of (i) nuclear fission (ii) nuclear fusion.
Answer:
(i) Nuclear fission is used in (1) a nuclear reactor as very efficient and the least-polluting source of energy to generate electricity (2) atomic bombs.
(ii) Nuclear fusion is used in (experimental) fusion reactors to generate electricity without the hazards of radioactive radiations and radioactive pollution which happens with fission reactors. Nuclear fusion reactions in the interior of stars are the source of their energy output and the means of synthesis of higher elements like carbon, nitrogen and silicon from hydrogen and helium.
Question 74.
Explain the basic exothermic reaction in stars.
Answer:
The fusion of hydrogen nuclei into helium nuclei results in release of energy. It is the basic exothermic reaction in stars.
(1) The proton-proton cycle :
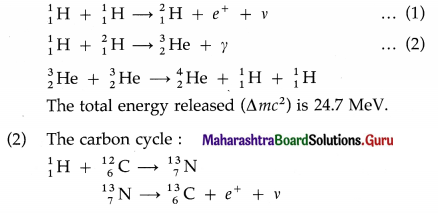
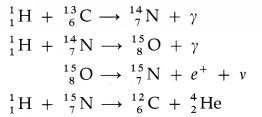
The total energy released is 24.7 MeV.
Question 75.
What is a nuclear reactor?
Answer:
A nuclear reactor is a device in which a nuclear fission chain reaction is used in a controlled manner (i) to produce energy in form of heat which is then converted into electricity or (ii) to produce radioisotopes or (iii) to produce new nuclides using a suitable fissionable material such as uranium or plutonium.
In a uranium reactor, \(\begin{gathered}
235 \\
92
\end{gathered}\)U is bombarded by slow neutrons to produce \(\begin{gathered}
235 \\
92
\end{gathered}\)U which undergoes fission.

Question 76.
What is a chain reaction? How is it produced?
Answer:
A chain reaction is a self-multiplying process in which neutrons ejected in a nuclear fission strike neighbouring nuclei of fissionable material and cause more fissions.
A fission of \(\begin{gathered}
235 \\
92
\end{gathered}\)U nucleus by a thermal neutron leads to ejection of two or three neutrons (2.7 neutrons on an average) having high kinetic energy of about 2 MeV. The kinetic energy of at least one of these neutrons is lowered to about 0.025 eV by a suitable moderator and the neutron is used to cause further fission. The process continues and hence it is called a chain reaction.
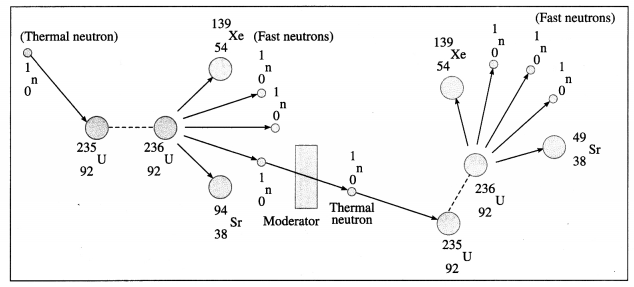
Multiple Choice Questions
Question 1.
The linear momentum of an electron in a Bohr orbit of an H-atom (principal quantum number n) is proportional to
(A) \(\frac{1}{n^{2}}\)
(B) \(\frac{1}{n}\)
(C) n
(D) n2.
Answer:
(B) \(\frac{1}{n}\)
Question 2.
The angular momenta of the electron in successive Bohr orbits differ by
(A) \(\frac{n h}{2 \pi}\)
(B) h
(C) \(\frac{h}{2 \pi}\)
(D) (n – 1)\(\frac{h}{2 \pi}\)
Answer:
(C) \(\frac{h}{2 \pi}\)
Question 3.
The angular momentum of the electron in the second Bohr orbit of hydrogen atom is l. Its angular moementum in the third Bohr orbit is
(A) \(\frac{2}{3}\) l
(B) \(\frac{3}{2}\) l
(C) 3l
(D) \(\frac{4}{3}\) l.
Answer:
(B) \(\frac{3}{2}\) l
Question 4.
The time taken by an electron moving with a speed of 2.18 × 106 m/s to complete one revolution in the first orbit (radius 0.53 A) of hydrogen atom is
(A) 1.527 × 10-15 s
(B) 1.527 × 10-16 s
(C) 1.527 × 10-17 s
(D) 1.527 × 10-18 s.
Answer:
(B) 1.527 × 10-16 s

Question 5.
If the electron in a hydrogen atom is raised to one of its excited energy states, the electron’s
(A) potential energy increases and kinetic energy decreases
(B) potential energy decreases and kinetic energy increases
(C) potential energy increases with no change in kinetic energy
(D) potential energy decreases but kinetic energy remains constant.
Answer:
(A) potential energy increases and kinetic energy decreases
Question 6.
The potential energy of the electron in a hydrogen atom in its ground state is
(A) – 6.8 eV
(B) – 13.6 eV
(C) – 27.2 eV
(D) 13.6 eV.
Answer:
(C) – 27.2 eV
Question 7.
The kinetic energy of the orbital electron in a hydrogen atom in the excited state corresponding to n = 2 is
(A) 3.4 eV
(B) 6.8 eV
(C) 13.6 eV
(D) 27.2 eV.
Answer:
(A) 3.4 eV
Question 8.
The ratio of the kinetic energy of an electron in a Bohr orbit to its total energy in the same orbit is
(A) -1
(B) 2
(C) \(\frac{1}{2}\)
(D) -0.5.
Answer:
(A) -1
Question 9.
The energy of an electron in the nth Bohr orbit is proportional to
(A) n2
(B) n
(C) \(\frac{1}{n}\)
(D) \(\frac{1}{n^{2}}\)
Answer:
(D) \(\frac{1}{n^{2}}\)

Question 10.
The radius of the first Bohr orbit is 0.53 Å and that of nth orbit is 212 Å. The value of n is
(A) 2
(B) 12
(C) 20
(D) 400.
Answer:
(C) 20
Question 11.
The radius and the energy of the first Bohr orbit in a hydrogen atom are r1 and E1. If the orbital electron makes a transition to a orbit of radius 4rv the energy of the electron changes to
(A) \(\frac{E_{1}}{4}\)
(B) \(\frac{E_{1}}{2}\)
(C) 2E1
(D) 4E1
Answer:
(A) \(\frac{E_{1}}{4}\)
Question 12.
A hydrogen atom in its ground state is excited to the state of energy E3 by an electron colliding with it. The minimum energy that the colliding electron must have is
(A) 10.2 eV
(B) 12.09 eV
(C) 12.5 eV
(D) 13.6 eV.
Answer:
(B) 12.09 eV
Question 13.
The energy of the electron in a hydrogen atom is raised from a state of energy E2 to that of energy E4. In the process, its
(A) energy doubles
(B) angular momentum doubles
(C) velocity doubles
(D) linear momentum doubles.
Answer:
(B) angular momentum doubles
Question 14.
Given that R is the Rydberg constant for hydrogen, the Hα line in the hydrogen spectrum has a wavelength
(A) \(\frac{1}{6 R}\)
(B) 6R
(C) \(\frac{5 R}{36}\)
(D) \(\frac{36}{5 R}\)
Answer:
(D) \(\frac{36}{5 R}\)

Question 15.
When the electron in a hydrogen atom jumps from the second orbit to the first orbit, the wavelength of the emitted rediation is λ. When the electron jumps from the third orbit to the first orbit, the wavelength of the emitted radiation would be
(A) \(\frac{27}{32}\) λ
(B) \(\frac{32}{27}\) λ
(C) \(\frac{2}{3}\) λ
(D) \(\frac{3}{2}\) λ.
Answer:
(A) \(\frac{27}{32}\) λ
Question 16.
In hydrogen atom, the Balmer series is obtained when the electron jumps from
(A) a higher orbit to the first orbit
(B) the first orbit to a higher orbit
(C) a higher orbit to the second orbit
(D) the second orbit to a higher orbit.
Answer:
(C) a higher orbit to the second orbit
Question 17.
The nuclei having the same number of protons but different number of neutrons are called
(A) isobars
(B) α-particles
(C) isotopes
(D) γ-particles.
Answer:
(C) isotopes
Question 18.
The nuclear volume of \(\begin{aligned}
&8 \\
&4
\end{aligned}\) Be is ………. that of \(\begin{aligned}
&1 \\
&1
\end{aligned}\) H.
(A) equal to
(B) two times
(C) four times
(D) eight times.
Answer:
(D) eight times.
Question 19.
The nuclear radius of the tungsten nuclide 74W is twice that of the sodium nuclide \(\begin{aligned}
&23 \\
&11
\end{aligned}\) Na. The neutron number of the tungsten nuclide is
(A) 82
(B) 100
(C) 110
(D) 184.
Answer:
(C) 110
Question 20.
When a β-particle is emitted by a nucleus, its mass number
(A) decreases
(B) remains the same
(C) increases
(D) may decrease or increase.
Answer:
(B) remains the same

Question 21.
When an α-particle is emitted by a nucleus, its mass number
(A) increases by 4
(B) decreases by 4
(C) increases by 2
(D) decreases by 2.
Answer:
(B) decreases by 4
Question 22.
When a γ-ray photon is emitted by an unstable nucleus,
(A) Z increases
(B) Z decreases
(C) A increases
(D) Z and A remain the same.
Answer:
(D) Z and A remain the same.
Question 23.
In a radioactive transformation, a change in the mass number occurs with
(A) α- or β-decay
(B) β-decay
(C) γ-decay
(D) α-decay.
Answer:
(D) α-decay.
Question 24.
The half-life of radium is 1600 y. How much of 1 μg of radium will remain undistintegrated after 8000 y?
(A) \(\frac{1}{8}\) μg
(B) \(\frac{1}{16}\) μg
(C) \(\frac{1}{32}\) μg
(D) \(\frac{1}{64}\) μg.
Answer:
(C) \(\frac{1}{32}\) μg
Question 25.
In one mean lifetime of a radioactive element, the fraction of the nuclei that has disintegrated is [e is the base of natral logarithm.]
(A) \(\frac{1}{e}\)
(B) 1 – \(\frac{1}{e}\)
(C) e
(D) e – 1.
Answer:
(B) 1 – \(\frac{1}{e}\)
Question 26.
The decay constant of a radioactive element is λ After a time 2λ-1 of the original number of radioactive nuclei about ……………….. remains undecayed.
(A) 37%
(B) 27%
(C) 25%
(D) 13.7%
Answer:
(D) 13.7%

Question 27.
Complete the following fission reaction :
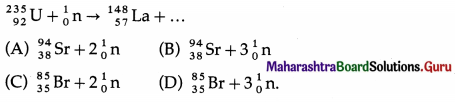
Answer:
(D) \({ }_{35}^{85} \mathrm{Br}+3{ }_{0}^{1} \mathrm{n}\)
Question 28.
The energy generated in the stars is because of
(A) radioactivity
(B) nuclear fission
(C) nuclear fusion
(D) photoelectric phenomenon.
Answer:
(C) nuclear fusion